This article has been reviewed according to Science X's editorial process and policies. Editors have highlighted the following attributes while ensuring the content's credibility:
fact-checked
trusted source
proofread
New research is helping scientists better understand how microstructures change
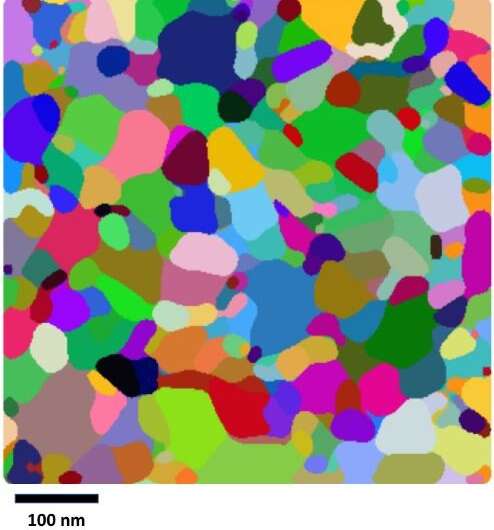
The orientations of these infinitesimally small separations between individual "grains" of a polycrystalline material have big effects. In a material such as aluminum, these collections of grains (called microstructures) determine properties such as hardness.
New research is helping scientists better understand how microstructures change, or undergo "grain growth," at high temperatures.
A team of materials scientists and applied mathematicians developed a mathematical model that more accurately describes such microstructures by integrating data that can be identified from highly magnified images taken during experiments. Their findings are published in Nature: Computational Materials.
The research team included Jeffrey M. Rickman, Class of '61 Professor of Materials Science & Engineering at Lehigh University; Katayun Barmak, Philips Electronics Professor of Applied Physics and Applied Mathematics at Columbia University; Yekaterina Epshteyn, Professor of Mathematics at the University of Utah; and Chun Liu, Professor of Applied Mathematics at the Illinois Institute of Technology.
"Our model is novel because it is given in terms of features that can be identified from experimental micrographs, or photos that reveal the details of microstructures at a length scale of nanometers to microns," Rickman said. "Because our model can be related to these experimental features, it is a more faithful representation of the actual grain growth process."
-
A grain-boundary network with disorientations highlighted. The grain-boundary network with associated boundary disorientations (color coded) and triple junctions for the micro-structure. Credit: Lehigh University -
The grain boundary segments with disorientations 50.0o ≤ φ ≤ 60.0o. Segments are color coded by length, with the longer segments shaded towards the red end ofthe color spectrum as indicated in the legend (by length in pixels). Credit: Lehigh University -
An automated trace of the grain boundaries and triple junctions corresponding to the microstructure. Credit: Courtesy of Lehigh University
The researchers conducted crystal orientation mapping on thin films of aluminum with columnar grains and used a stochastic, marked point process to represent triple junctions, points where three grains and grain boundaries meet in the structure. Their model is the first to integrate data on the interactions and disorientations of these triple junctions to predict grain growth.
Predicting grain growth is key to the creation of new materials and is a pivotal area of study in materials science. As a result, many models of grain growth have been developed. However, the project's direct link between the mathematical model and the experimental micrographs is highly distinctive.
According to Rickman, linking the model directly to features that can be tracked during experiments will benefit computational materials scientists who model the kinetics of grain growth.
"Ultimately, this research provides a way to better understand how grain growth works and how it can be used to inform the development of new materials," Rickman said.
More information: J. M. Rickman et al, Point process microstructural model of metallic thin films with implications for coarsening, npj Computational Materials (2023). DOI: 10.1038/s41524-023-00986-w
Provided by Lehigh University